Abstract
Jasmonates induce the protein–protein interaction between the F-box protein CORONATINE INSENSITIVE 1 (COI1) and jasmonate ZIM-domain proteins (JAZs) in the presence of inositol phosphate, which made the degradation of JAZs and the release of the JAZ-repressed transcription factors. They are involved in the regulation of a wide range of physiology process, including plant growth, development and stress response. Coronatine-O-methyloxime (COR-MO) prevents the binding of COI1-JAZ, acting as an antagonist for jasmonate signaling pathway, while the understanding on the molecular basis of its action as an antagonist is still lacking at atomic level. In this study, we explored the interaction mechanism of jasmonate antagonists through molecular docking, molecular dynamics (MD) simulation, residue interaction network analysis and binding free energy calculation. Compared with the agonists, the conformation of JAZ1 is different in response to the binding with antagonist. Antagonists lost hydrogen bond interaction with Ala204 and Arg206 in JAZ1, and Arg496 in COI1, which results that the sidechain of Arg206 in JAZ1 rotates and unable to penetrate into COI1, so that it lost interaction with 1,5-InsP8. It is indicated that the agonist is more closely associated with 1,5-InsP8 than the antagonist based on the residue interaction network analysis. The binding free energy of JA-Ile-MO/COR-MO with JAZ1 is higher than that of JA-Ile/COR. It is unfavorable for the binding of JAZ1 with COI1 in the presence of antagonists. This study provides a basis for the understanding of the interaction mechanism of jasmonate agonists/antagonists, which will contribute to the discovery of novel jasmonate agonists/antagonists.









Similar content being viewed by others
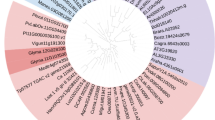
Data availability
The datasets generated during and/or analysed during the current study are available from the corresponding author on reasonable request.
References
Browse J (2008) Jasmonate passes muster: a receptor and targets for the defense hormone. Annu Rev Plant Biol 60:183–205. https://doi.org/10.1146/annurev.arplant.043008.092007
Chini A, Fonseca S, Fernández G, Adie B, Chico JM et al (2007) The JAZ family of repressors is the missing link in jasmonate signalling. Nature 448:666–671. https://doi.org/10.1038/nature06006
Sheard LB, Tan X, Mao H, Withers J, Ben-Nissan G et al (2010) Jasmonate perception by inositol-phosphate-potentiated COI1-JAZ co-receptor. Nature 468:400–407. https://doi.org/10.1038/nature09430
Katsir L, Schilmiller AL, Staswick PE, He SY, Howe GA (2008) COI1 is a critical component of a receptor for jasmonate and the bacterial virulence factor coronatine. Proc Natl Acad Sci USA 105:7100–7105. https://doi.org/10.1073/pnas.0802332105
Thines B, Katsir L, Melotto M, Niu Y, Mandaokar A et al (2007) JAZ repressor proteins are targets of the SCF(COI1) complex during jasmonate signalling. Nature 448:661–665. https://doi.org/10.1038/nature05960
Chini A, Gimenez-Ibanez S, Goossens A, Solano R (2016) Redundancy and specificity in jasmonate signalling. Curr Opin Plant Biol 33:147–156. https://doi.org/10.1016/j.pbi.2016.07.005
Zhang F, Ke J, Zhang L, Chen R, Sugimoto K et al (2017) Structural insights into alternative splicing-mediated desensitization of jasmonate signaling. Proc Natl Acad Sci USA 114:1720–1725. https://doi.org/10.1073/pnas.1616938114
Thireault C, Shyu C, Yoshida Y, St. Aubin B, Campos ML et al (2015) Repression of jasmonate signaling by a non-TIFY JAZ protein in Arabidopsis. Plant J 82:669–679. https://doi.org/10.1111/tpj.12841
Howe GA, Major IT, Koo AJ (2018) Modularity in jasmonate signaling for multistress resilience. Annu Rev Plant Biol 69:387–415. https://doi.org/10.1146/annurev-arplant-042817-040047
Takaoka Y, Nagumo K, Azizah IN, Oura S, Iwahashi M et al (2019) A comprehensive in vitro fluorescence anisotropy assay system for screening ligands of the jasmonate COI1–JAZ co-receptor in plants. J Biol Chem 294:5074–5081. https://doi.org/10.1074/jbc.RA118.006639
Wasternack C, Hause B (2013) Jasmonates: biosynthesis, perception, signal transduction and action in plant stress response, growth and development. An update to the 2007 review in Annals of Botany. Ann Bot 111:1021–1058. https://doi.org/10.1093/aob/mct067
Monte I, Hamberg M, Chini A, Gimenez-Ibanez S, García-Casado G et al (2014) Rational design of a ligand-based antagonist of jasmonate perception. Nat Chem Biol 10:671–676. https://doi.org/10.1038/nchembio.1575
Takaoka Y, Iwahashi M, Chini A (2018) A rationally designed JAZ subtype-selective agonist of jasmonate perception. Nat Commun 9:3654. https://doi.org/10.1038/s41467-018-06135-y
Xie D-X, Feys BF, James S, Nieto-Rostro M, Turner JG (1998) COI1: an arabidopsis gene required for jasmonate-regulated defense and fertility. Science 280:1091–1094. https://doi.org/10.1126/science.280.5366.1091
Bender CL, Alarcón-Chaidez F, Gross DC (1999) Pseudomonas syringae phytotoxins:mode of action, regulation, and biosynthesis by peptide and polyketide synthetases. Microbiol Mol Biol Rev 63:266–292. https://doi.org/10.1128/MMBR.63.2.266-292.1999
Fonseca S, Chini A, Hamberg M, Adie B, Porzel A et al (2009) (+)-7-iso-Jasmonoyl-L-isoleucine is the endogenous bioactive jasmonate. Nat Chem Biol 5:344–350. https://doi.org/10.1038/nchembio.161
Melotto M, Mecey C, Niu Y, Chung HS, Katsir L et al (2008) A critical role of two positively charged amino acids in the Jas motif of Arabidopsis JAZ proteins in mediating coronatine- and jasmonoyl isoleucine-dependent interactions with the COI1 F-box protein. Plant J 55:979–988. https://doi.org/10.1111/j.1365-313x.2008.03566.x
Yan J, Zhang C, Gu M, Bai Z, Zhang W et al (2009) The Arabidopsis CORONATINE INSENSITIVE1 protein is a jasmonate receptor. Plant Cell 21:2220–2236. https://doi.org/10.1105/tpc.109.065730
Zhang L, Yao J, Withers J, Xin X-F, Banerjee R et al (2015) Host target modification as a strategy to counter pathogen hijacking of the jasmonate hormone receptor. Proc Natl Acad Sci USA 112:14354–14359. https://doi.org/10.1073/pnas.1510745112
Yan J, Li S, Gu M, Yao R, Li Y et al (2016) Endogenous bioactive jasmonate is composed of a set of (+)-7-iso-JA-amino acid conjugates. Plant Physiol 172:2154–2164. https://doi.org/10.1104/pp.16.00906
Littleson MM, Baker CM, Dalençon AJ, Frye EC, Jamieson C et al (2018) Scalable total synthesis and comprehensive structure–activity relationship studies of the phytotoxin coronatine. Nat Commun 9:1105. https://doi.org/10.1038/s41467-018-03443-1
Scherzer S, Shabala L, Hedrich B, Fromm J, Bauer H et al (2017) Insect haptoelectrical stimulation of Venus flytrap triggers exocytosis in gland cells. Proc Natl Acad Sci USA 114:4822–4827. https://doi.org/10.1073/pnas.1701860114
Cui MQ, Du J, Yao XJ (2018) The binding mechanism between inositol phosphate (InsP) and the jasmonate receptor complex: a computational study. Front Plant Sci. https://doi.org/10.3389/fpls.2018.00963
Valenzuela-Riffo F, Garrido-Bigotes A, Figueroa PM, Morales-Quintana L, Figueroa CR (2018) Structural analysis of the woodland strawberry COI1-JAZ1 co-receptor for the plant hormone jasmonoyl-isoleucine. J Mol Graph Model 85:250–261. https://doi.org/10.1016/j.jmgm.2018.09.004
Garrido-Bigotes A, Valenzuela-Riffo F, Torrejón M, Solano R, Morales-Quintana L et al (2020) A new functional JAZ degron sequence in strawberry JAZ1 revealed by structural and interaction studies on the COI1–JA-Ile/COR–JAZs complexes. Sci Rep 10:11310. https://doi.org/10.1038/s41598-020-68213-w
Saito R, Hayashi K, Nomoto H, Nakayama M, Takaoka Y et al (2021) Extended JAZ degron sequence for plant hormone binding in jasmonate co-receptor of tomato SlCOI1-SlJAZ. Sci Rep 11:13612–13612. https://doi.org/10.1038/s41598-021-93067-1
Wang L, Wu Y, Deng Y, Kim B, Pierce L et al (2015) Accurate and reliable prediction of relative ligand binding potency in prospective drug discovery by way of a modern free-energy calculation protocol and force field. J Am Chem Soc 137:2695–2703. https://doi.org/10.1021/ja512751q
Saha A, Shih AY, Mirzadegan T, Seierstad M (2018) Predicting the binding of fatty acid amide hydrolase inhibitors by free energy perturbation. J Chem Theory Comput 14:5815–5822. https://doi.org/10.1021/acs.jctc.8b00672
Li ZD, Zhang JZH (2021) Quantitative analysis of ACE2 binding to coronavirus spike proteins: SARS-CoV-2 vs. SARS-CoV and RaTG13. Phys Chem Chem Phys 23:13926–13933. https://doi.org/10.1039/D1CP01075A
Zhang YZ, He XB, Zhai JC, Ji BH, Man VH et al (2021) In silico binding profile characterization of SARS-CoV-2 spike protein and its mutants bound to human ACE2 receptor. Brief Bioinform. https://doi.org/10.1093/bib/bbab188
Vanga SR, Åqvist J, Hallberg A, Gutiérrez-de-Terán H (2021) Structural basis of inhibition of human insulin-regulated aminopeptidase (IRAP) by Benzopyran-based inhibitors. Front Mol Biosci 8:625274. https://doi.org/10.3389/fmolb.2021.625274
Cui M, Du J, Yao X (2018) The binding mechanism between inositol phosphate (InsP) and the jasmonate receptor complex: a computational study. Front Plant Sci 9:963. https://doi.org/10.3389/fpls.2018.00963
Wang H, Falck JR, Hall TMT, Shears SB (2011) Structural basis for an inositol pyrophosphate kinase surmounting phosphate crowding. Nat Chem Biol 8:111–116. https://doi.org/10.1038/nchembio.733
Freeman LC (1978) Centrality in social networks conceptual clarification. Soc Netw 1:215–239
Frisch MJ, Trucks GW, Schlegel HB, Scuseria GE, Robb MA et al (2009) Gaussian Inc., Wallingford
Sastry GM, Adzhigirey M, Day T, Annabhimoju R, Sherman W (2013) Protein and ligand preparation: parameters, protocols, and influence on virtual screening enrichments. J Comput Aid Mol Des 27:221–234. https://doi.org/10.1007/s10822-013-9644-8
Friesner RA, Banks JL, Murphy RB, Halgren TA, Klicic JJ et al (2004) Glide: a new approach for rapid, accurate docking and scoring. 1. Method and assessment of docking accuracy. J Med Chem 47:1739–1749. https://doi.org/10.1021/jm0306430
Schrödinger LLC ( 2010) The PyMOL molecular graphics system, Version 1.3.1
Zoete V, Cuendet MA, Grosdidier A, Michielin O (2011) SwissParam: a fast force field generation tool for small organic molecules. J Comput Chem 32:2359–2368. https://doi.org/10.1002/jcc.21816
Gordon JC, Myers JB, Folta T, Shoja V, Heath LS et al (2005) H++: a server for estimating pKas and adding missing hydrogens to macromolecules. Nucleic Acids Res 33:368–371. https://doi.org/10.1093/nar/gki464
Phillips JC, Braun R, Wang W, Gumbart J, Tajkhorshid E et al (2005) Scalable molecular dynamics with NAMD. J Comput Chem 26:1781–1802. https://doi.org/10.1002/jcc.20289
Mackerell AD Jr, Feig M, Brooks CL 3rd (2004) Extending the treatment of backbone energetics in protein force fields: limitations of gas-phase quantum mechanics in reproducing protein conformational distributions in molecular dynamics simulations. J Comput Chem 25:1400–1415. https://doi.org/10.1002/jcc.20065
Coleman TG, Mesick HC, Darby RL (1977) Numerical integration: a method for improving solution stability in models of the circulation. Ann Biomed Eng 5:322–328. https://doi.org/10.1056/NEJM193212292072608
Tom D, Darrin Y, Lee P (1993) Particle mesh Ewald: an N [center-dot] log(N) method for Ewald sums in large systems. J Chem Phys 98:10089–10092
Piovesan D, Minervini G, Tosatto SCE (2016) The RING 2.0 web server for high quality residue interaction networks. Nucleic Acids Res 44:W367–W374. https://doi.org/10.1093/nar/gkw315
Martin AJ, Vidotto M, Boscariol F, Di DT, Walsh I et al (2011) RING: networking interacting residues, evolutionary information and energetics in protein structures. Bioinformatics 27:2003–2005. https://doi.org/10.1093/bioinformatics/btr191
Doncheva NT, Klein K, Domingues FS, Albrecht M (2011) Analyzing and visualizing residue networks of protein structures. Trends Biochem Sci 36:179–182. https://doi.org/10.1016/j.tibs.2011.01.002
Shannon P, Markiel A, Ozier O, Baliga NS, Wang JT et al (2003) Cytoscape: a software environment for integrated models of biomolecular interaction networks. Routledge 13:2498–2504. https://doi.org/10.3372/wi.43.43119
Wan S, Bhati AP, Zasada SJ, Coveney PV (2020) Rapid, accurate, precise and reproducible ligand–protein binding free energy prediction. Interface Focus 10:20200007. https://doi.org/10.1098/rsfs.2020.0007
(2005) Prime version 2.1. Schrödinger LLC, New York
Shivakumar D, Williams J, Wu Y, Damm W, Shelley J et al (2010) Prediction of absolute solvation free energies using molecular dynamics free energy perturbation and the OPLS force field. J Chem Theory Comput 79:961–967. https://doi.org/10.1021/ct900587b
Jianing L, Robert A, Kai Z, Yixiang C, Suwen Z et al (2011) The VSGB 2.0 model: a next generation energy model for high resolution protein structure modeling. Proteins 79:2794–2812. https://doi.org/10.1002/prot.23106
Humphrey W, Dalke A, Schulten K (1996) VMD: visual molecular dynamics. J Mol Graph 14:33–38. https://doi.org/10.1016/0263-7855(96)00018-5
Jimenez-Aleman GH, Almeida-Trapp M, Fernández-Barbero G, Gimenez-Ibanez S, Reichelt M et al (2019) Omega hydroxylated JA-Ile is an endogenous bioactive jasmonate that signals through the canonical jasmonate signaling pathway. BBA-Mol Cell Biol L 1864:158520. https://doi.org/10.1016/j.bbalip.2019.158520
Poudel AN, Holtsclaw RE, Kimberlin A, Sen S, Zeng S et al (2019) 12-Hydroxy-jasmonoyl-l-isoleucine is an active jasmonate that signals through CORONATINE INSENSITIVE 1 and contributes to the wound response in arabidopsis. Plant Cell Physiol 60:2152–2166. https://doi.org/10.1093/pcp/pcz109
Laha D, Johnen P, Azevedo C, Dynowski M, Weiß M et al (2015) VIH2 regulates the synthesis of inositol pyrophosphate InsP8 and jasmonate-dependent defenses in arabidopsis. Plant Cell 27:1082–1097. https://doi.org/10.1105/tpc.114.135160
Laha D, Parvin N, Dynowski M, Johnen P, Mao H et al (2016) Inositol polyphosphate binding specificity of the jasmonate receptor complex. Plant Physiol 171:2364–2370. https://doi.org/10.1104/pp.16.00694
Yan J, Yao R, Chen L, Li S, Gu M et al (2018) Dynamic perception of jasmonates by the F-box protein COI1. Mol Plant 11:1237–1247. https://doi.org/10.1016/j.molp.2018.07.007
Acknowledgements
This work was funded by the Natural Science Foundation of Shandong Province, China (Grant No. ZR2018QB004), the Talents of High Level Scientific Research Foundation (Grant No. 6631113318) of Qingdao Agricultural University, and the National Natural Science Foundation of China (Grant No. 81903748). We are thankful for the software provided by professor Xiaojun Yao at Lanzhou university.
Author information
Authors and Affiliations
Contributions
JD conceived and supervised the experiments. MQC, KZ, and RHW performed MD simulations. MQC, KZ, and RHW analyzed the data. MQC and JD wrote the paper.
Corresponding author
Ethics declarations
Conflict of interest
The authors declare that there are no conflicts of interest.
Additional information
Publisher's Note
Springer Nature remains neutral with regard to jurisdictional claims in published maps and institutional affiliations.
Supplementary Information
Below is the link to the electronic supplementary material.
10822_2022_441_MOESM2_ESM.tif
Supplementary file2 (TIF 74 kb). Figure S1 The total number of H-bonds formed between ligand and the receptor. (A) system 1, COI1+ASK1+JAZ1+1,5-InsP8+JA-Ile-MO, (B) system 2, COI1+ASK1+JAZ1+1,5-InsP8+COR-MO, (C) system 3, COI1+ASK1+JAZ1+1,5InsP8+JA-Ile, (D) system 4, COI1+ASK1+JAZ1+1,5-InsP8+COR. (E) The occupancy of hydrogen bond formed between ligand and the receptor
10822_2022_441_MOESM3_ESM.tif
Supplementary file3 (TIF 404 kb). Figure S2 The interaction of COR-MO/COR (sphere representation) with COI1 (gray; ribbon representation), JAZ1 degron (yellow; surface representation) and 1,5-InsP8 (sphere representation). (A) system 2, COI1+ASK1+JAZ1+1,5-InsP8+COR-MO; (B) system 4, COI1+ASK1+JAZ1+1,5-InsP8+COR
Rights and permissions
About this article
Cite this article
Cui, M., Zhang, K., Wu, R. et al. Exploring the interaction mechanism between antagonist and the jasmonate receptor complex by molecular dynamics simulation. J Comput Aided Mol Des 36, 141–155 (2022). https://doi.org/10.1007/s10822-022-00441-w
Received:
Accepted:
Published:
Issue Date:
DOI: https://doi.org/10.1007/s10822-022-00441-w